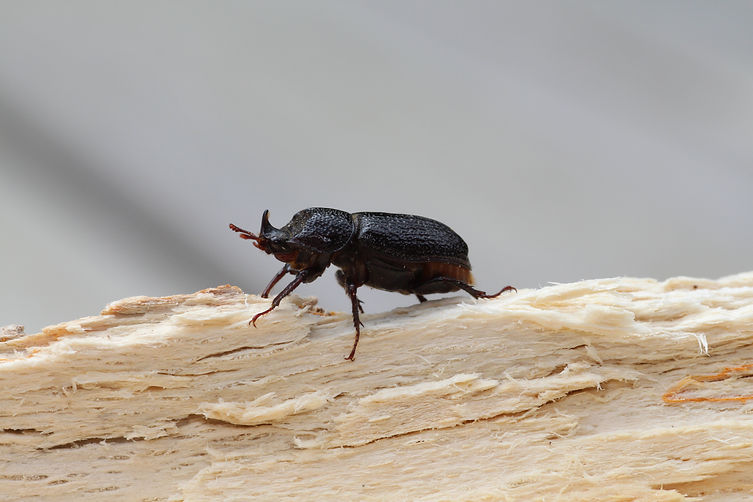
Talk abstracts
Abstracts for scheduled talks can be found below:
Neurogenomic mechanisms of social plasticity
Rui F. Oliveira (ruiol@ispa.pt), ISPA – Instituto Universitário, Lisboa, Portugal
Group-living animals must adjust the expression of their social behaviour to changes in their social environment and to transitions between life-history stages, and this social plasticity can be seen as an adaptive trait that can be under positive selection when changes in the environment outpace the rate of genetic evolutionary change. Here, I will propose a conceptual framework for understanding the neuromolecular mechanisms of social plasticity. According to this framework, social plasticity is achieved by rewiring or by biochemically switching nodes of a neural network underlying social behaviour in response to perceived social information. Therefore, at the molecular level, it depends on the social regulation of gene expression, so that different genomic and epigenetic states of this brain network correspond to different behavioural states, and the switches between states are orchestrated by signalling pathways that interface the social environment and the genotype. Different types of social plasticity can be recognized based on the observed patterns of inter- versus intra-individual occurrence, time scale and reversibility. It is proposed that these different types of social plasticity rely on different proximate mechanisms at the physiological, neural and genomic level.
Honey bee aggression and brain energy metabolism: a plasticity mechanism that stands the test of time
Clare Rittschof (clare.rittschof@uky.edu), University of Kentucky, Lexington, USA
Socially regulated behavioral plasticity is essential to societal function in the honey bee (Apis mellifera). Honey bee workers behave aggressively in the context of anti-predator defense, an event that involves hundreds of individuals, specialized worker castes, and alarm pheromone signaling. Predator threats and social cues induce a rapid aggressive response and also modulate aggression level over hours or days. Using transcriptomic and metabolomics data, as well as pharmacological and genetic manipulations, we showed that high aggression is associated with a brain energy metabolic phenotype known as aerobic glycolysis. This relationship is largely consistent across the many contexts and time scales for variation in aggression. Aerobic glycolysis appears to cause an enhanced response to future threats, and the pattern is conserved in other animal species. Moreover, nutritional manipulations that modulate brain mitochondrial function in other species also cause a change in honey bee aggression, suggesting that many intrinsic and extrinsic factors utilize a similar mechanism to cause behavioral change. Currently we are evaluating the functional role of aerobic glycolysis in the aggressive brain, and examining how this single mechanism regulates aggression with variable degrees of plasticity. Honey bee aggression offers a unique opportunity to evaluate plasticity in behavior at different time scales.
Plasticity is not a trait
Alastair Wilson (A.Wilson@exeter.ac.uk), University of Exeter, Penryn, UK
Over the last decade, behavioural ecologists have increasingly viewed phenotypic plasticity “as a trait” to be studied under an adaptationist paradigm. Frequently reaction norms models are used where a trait (y) is assumed to be a (typically) linear function of the environment (E), with a slope interpretable as plasticity (p). Simple and intuitive, reaction norms also offer statistical benefits for empiricists with limited data. However, these benefits come with costs and pitfalls that need to be recognised. First, while plasticity can be adaptive, proving this is challenging (and more is probably maladaptive). Second, while p can be viewed as under selection, it is often better understood as the cause of selection on y. Third, analysing one component (p) of a bivariate phenotype (the reaction norm also has an intercept) will generate erroneous conclusions. Fourth, strong dependence of model parameters on (arbitrary) scaling and centering of E makes it easy for unwary researchers to misinterpret analyses. Fortunately, most pitfalls can be readily avoided through better understanding of the relationship between reaction norms and “character state” models. Here I highlight some equivalences (and differences) between these two approaches and suggest that wider use of the latter would benefit plasticity research.
Understanding the “how” of behavioural plasticity using Drosophila melanogaster sperm competition responses as a model
Amanda Bretman (a.j.bretman@leeds.ac.uk), University of Leeds, Leeds, UK
Behavioural plasticity requires animals to monitor and integrate transient environmental cues, and then accurately adjust their strategy, not just in terms of whether or not to produce a behaviour, but also the level of production. Therefore, it is likely to be mechanistically very complex. Classically, behavioural ecology has been excellent at investigating the “why” of such plastic behaviours, and now technology is making it possible to tackle the “how” at various biological levels. Here the fruit fly Drosophila melanogaster continues to be one of our most powerful tools. As in many species, male D. melanogaster can reap fitness benefits by tracking their sperm competitive environment and altering their reproductive investment accordingly. Specifically, they increase their mating duration and ejaculate components if exposed to another male, and reduce these when the male is removed. Using this extremely tractable model, we have begun to dissect how males are able to do this, exploring the mechanisms linking sensory inputs to behavioural outputs. By integrating genetic, physiological, neural and evolutionary approaches we can gain deep insights into the coordination of processes governing behavioural plasticity. Ultimately such approaches can help us to understand the opportunities for, and constraints on, plasticity generally.
Social learning, the spread of innovation and a changing world - studies in great tits (Parus major)
Lucy Aplin (lucy.aplin@zoo.ox.ac.uk), University of Oxford, Oxford, UK
In many species, learning by experience is a vital form of plasticity that allows individuals to fine-tune their behaviour to local environmental conditions. In addition, animals can increase their behavioural repertoire through the innovation of novel skills or behaviours. Such innovativeness may enable individuals to exploit modified environments, and is therefore of particular interest under human-induced rapid environmental change. While innovations may be rare events, the acquisition of behaviours via social learning can allow innovations spread between individuals and through populations. Social learning thus a vital, but often understudied, source of behavioural plasticity. The social transmission of information is a multi-faceted process requiring consideration of ecology, cognition and social structure. Perhaps the most famous example of the spread and persistence of an innovation in non-human animals occurred in tits (parids), when individuals began piercing milk bottles to access cream. I will review studies of innovations and social learning in parids. By doing so I will address three questions: 1) what are the predictors of individual variation in innovativeness and social learning? 2) how do social dynamics influence the diffusion of innovations? 3) what might be the evolutionary significance of socially learned innovations?
Does transgenerational plasticity increase robustness to changes in environmental variability?
​Bram Kuijper (a.kuijper@ucl.ac.uk), UCL, London & University of Exeter, Penryn, UK
Recent studies predict that transgenerational plasticity via parental effects can be adaptive in fluctuating environments, as it allows parents to inform offspring about conditions they are likely to face. However, these predictions only consider environments of constant spatial or temporal variability, whereas studies on climate change show that environmental variability is, in fact, rarely constant. To this end, I use a model to predict whether evolving transgenerational plasticity increases robustness to various changes in environmental variability. Throughout, I find that populations which originally experience slowly fluctuating environments are much more robust to new environmental changes, relative to populations that originally experience rapidly fluctuating environments. This is striking, as both populations initially evolve the same magnitude of transgenerational plasticity. The key difference is that transgenerational plasticity in a slowly changing environment leads to positive parent-offspring correlations, which enhance an evolutionary response to novel environments. By contrast, rapidly changing environments result in negative parent-offspring correlations, which hamper a rapid evolutionary response to change.